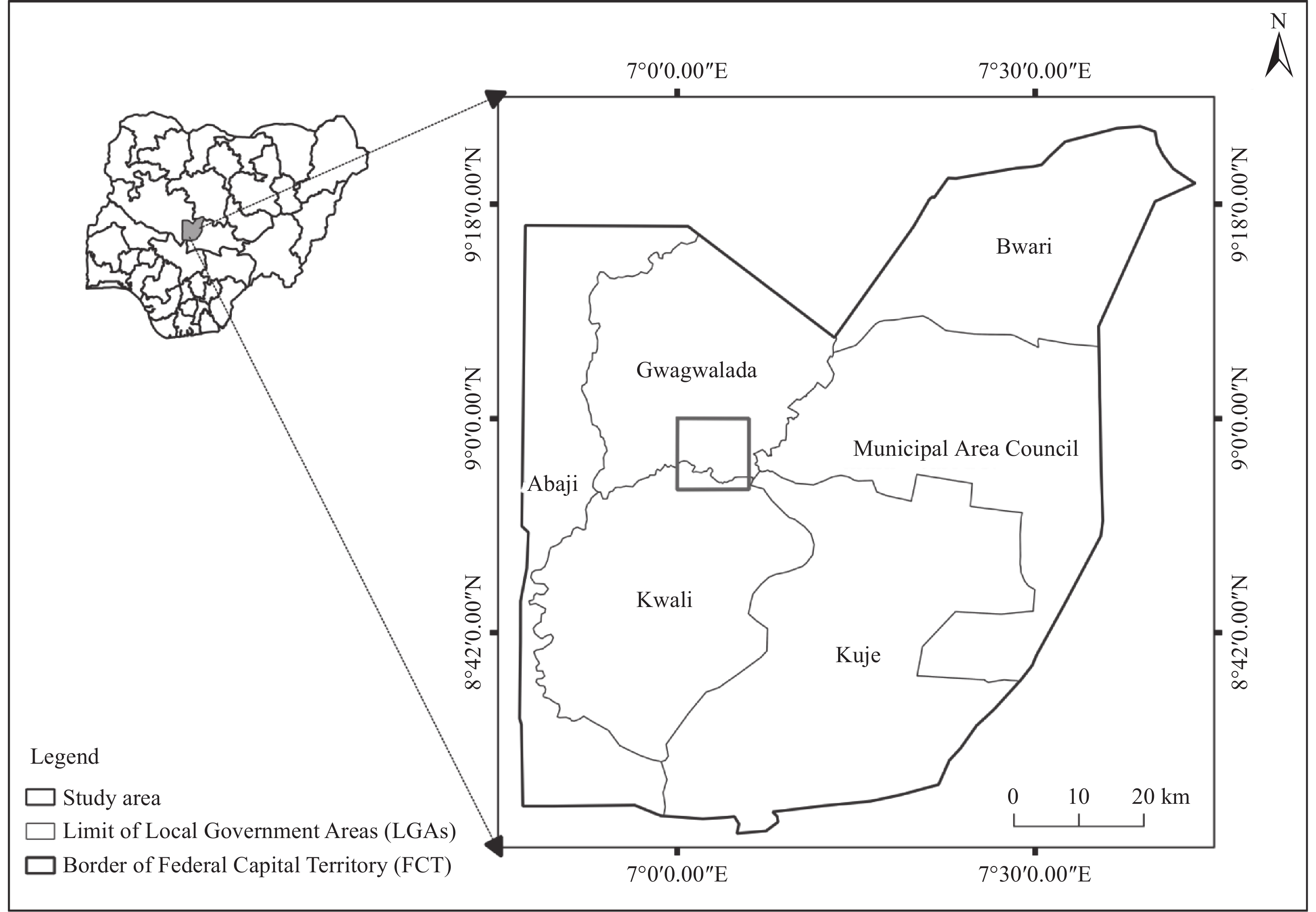
Citation: | ILUNGA N, AMADI AN, NDATIMANA G, et al. 2024. Evaluation of aquifer hydraulic properties from resistivity and pumping test data in parts of Gwagwalada, Northcentral Nigeria. Journal of Groundwater Science and Engineering, 12(3): 309-320 doi: 10.26599/JGSE.2024.9280023 |
Freshwater, made up of surface water (rivers, streams and lakes) and groundwater (Mishra, 2023), is the main source of water for various human activities such as agriculture, domestic use, fisheries among others (Gleick, 1996). Indeed, surface water resources are the most efficient way to meet water demand on planet (Van der Meulen et al. 2020). However, freshwater resource is unevenly distributed around the world (Khilchevskyi and Karamushka, 2021), hence not readily available to all (Nazario et al. 2023; Kasidi, 2017).
Federal Capital Territory (FCT) Abuja is underlain by the basement complex (Sunkari et al. 2021; Dinneya and Adigun, 2022) where surface sources of water supply are either inadequate, intermittent or polluted (Kasidi, 2017; Ighalo and Adeniyi, 2020). Consequently, domestic and industrial supplies depend heavily on groundwaters (Okogbue and Omonona, 2013; Rojanasakul et al. 2023). Evidently, groundwater is the main source of potable water supply in Abuja (Omada et al. 2024), supplementing the surface water which had become inadequate in both quality and quantity (Okogbue and Omonona, 2013; Umar et al. 2019). Nevertheless, this water source does not find everywhere in FCT (Sunkari et al. 2021), neither the yields of boreholes within the geological zones is efficient (Olasehinde et al. 2016). Notably, many folks are in dire need of this scare resources (Asije and Igwe, 2014; Sunkari et al. 2021). Moreover, water resources' need increase as the population density and economy of metropolitan cities especially in developing countries (e.g. FCT) increase (Nugraha et al. 2022; Nazari and Keshavarz, 2023). Unfortunately, such drastic increase in population goes hand in hand with the continuous contamination of surface waters (Lapworth et al. 2022) and shallow aquifers. Gwagwalada area of Abuja is at the centre of the upsurge in population (Achichi et al. 2023) owing to (1) its location in the Federal Capital of Nigeria, (2) presence of the university and teaching hospital, (3) as well as mining, (4) agricultural and (5) industrial activities (Etu-Efeotor, 1998). Consequently, this has led to increase in the demand for groundwater to meet the water need of the people in the area (Dan-hassan et al. 2012).
There are several techniques and methods to assess groundwater, such as geophysical (Olasehinde, 1999; Hazell et al. 1992; Wiederhold et al. 2021), geological (Rizwan, 2018; Chilton and Forester, 1993; Haque et al. 2020), geospatial (Adewumi and Anifowose, 2017; Pal et al. 2020), and pumping test methods (Olorunfemi and Fasuyi, 1993; Amadi et al. 2020; Hasan et al. 2021). However, there is scanty information on the use of resistivity methods in Gwagwalada area, FCT. Thus, the current research aimed at evaluating the aquifer hydraulic properties in parts of Gwagwalada through resistivity method by computing Dar-Zarrouk parameters and pumping tests. Vertical Electrical Sounding (VES) techniques are widely used to obtain Aquifer parameters (Olayinka, 1990; Dan-Hassan and Yaya, 2007; Nugraha et al. 2022). Dar-Zarrouk parameters consists of longitudinal conductance, S (Ω−1) and Transverse resistance, T (Ωm2) (Henriet, 1976; Egbai and Iserhien-Emekeme, 2015; Naidu et al. 2021; Mahmud et al. 2022) and are derived from resistivity values (Egbai and Iserhien-Emekeme, 2015; Yusuf et al. 2021). Aquifer test is the most suitable means for estimating hydraulic characteristics (Fetter, 2001; Dan-hassan, 2013), since digests field data that reflects not only the point behavior of well location but also a certain volume of aquifer material around the well within the cone of depression (Sen, 2015).
In the current research, the groundwater potential map was drawn up based on hydraulic properties, namely transmissivity and hydraulic conductivity. The findings are expected to shed light on understanding of the aquifer systems and their properties to ensure sustainability of dependent life in Gwagwalada, especially the community in dire need of this water source. This would undoubtedly be achieved through the use of established groundwater potential and aquifer protective capacity maps of parts of Gwagwalada, the useful tools for effective future groundwater exploitation in this area.
The study area is situated in the South-West of Gwagwalada, which is a part of FCT Abuja in Nigeria, lying between latitude 8°54ʹ00ʺ to 9°00ʹ00ʺ N and longitude 7°00ʹ00ʺ to 07°06ʹ00ʺ E (Fig. 1). The communities within the study area include Dukpa, Deshi Paiko, Paiko, Passu, Korokola, Kaida, Checheyi, Bako and Gwagwalada. The area is easily accessible due to a good road network.
Abuja is characterized by a well-defined dry and rainy season; the rainy season falls between April and October while the dry season is between Novembers to March. The rainy season is characterized by rise in water table and most streams have water while during the dry season, most of these streams are dried up. Federal Capital Territory, Abuja consists of 85% igneous and high-grade metamorphic rocks of the Nigerian basement complex and Cretaceous sedimentary rocks of the Bida Basin, which cover the remaining 15%. These rocks consist of gneiss, granite-gneiss and granites (Fig. 2) and are generally oriented from North-Northeast-South-Southwest (NNE-SSW). In most cases the rocks have weathered into reddish sandy clay to clay materials capped by laterite with lots of mica flakes (Offodile, 1992). In the study area, granite-gneiss are well represented among the three aforementioned major rock categories. The structural pattern in the area is characterized by two joint directions, NE-SW as the major, then the SE-NW direction; implying that the area has witnessed two orogenic events that are different in time and direction.
Electrical resistivity surveying involving Vertical Electrical Soundings (VES) was carried out in this research. As such, ten VES measurements were employed within the study area using the Schlumberger array configuration, chosen preferably by the side of the boreholes subjected to pumping test. The electrical resistivity measurements were performed using the ABEM SAS Terrameter 1000 powered by 12V D.C power source. For the current injection and voltage record, four cable wheels and four electrodes were used, while, four hammers and a measuring tape were used for fixing the electrodes and their separation measurements respectively. Current electrode separation, AB/2, and potential electrode, MN/2, were ranged respectively from 1 meter to a maximum of 160 meters and from 0.5 meter to 20 meters. The resistivity data was obtained by injecting the current electrodes (A and B) into the ground, thus the voltage difference between potential electrodes (M and N) was determined. Based on voltage (V) and current (I) values obtained, the apparent resistivity (ρa) was calculated as follows:
(1) |
The resistivity survey helped to determine the subsurface conditions of the area. VES data were interpreted using the curve matching technique. The curves were obtained by plotting the apparent resistivity data (ρa) as ordinates against the electrode AB spacing as abscissa in the log-log plot through the computer iteration using WINRESIST software. The subsurface conditions were deduced from a measured sounding curve in terms of the number of layers, their resistivities and thicknesses. Resistivities and thicknesses of each geoelectric layers are combined together to obtain the Dar Zarrouk parameters (Yusuf et al. 2021; Egbai and Iserhien-Emekeme, 2015), consists of Longitudinal conductance, S (Ω−1) and Transverse resistance, Tr (Ωm2) (Egbai and Iserhien-Emekeme, 2015). For a sequence of n layers of resistivity ρi and thickness hi, the longitudinal unit conductance, S and the transverse unit resistance, T are defined by:
(2) |
(3) |
Where: S is the longitudinal conductance (Ω−1); Tr is the transverse resistance (Ωm2)
The protective capacity and longitudinal unit conductance are considered to have a proportional relationship. Longitudinal unit conductance, S can be used directly in the protective capacity evaluation of aquifers to signify the regulation of percolation of contaminants into the aquifer (Henriet, 1976; Yusuf and Abiye, 2019).
The determination of the groundwater flow direction involved the measurement of Static Water Level (SWL) from 30 existing hand dug wells, their topographic elevations and coordinates in nearby communities within the study area. The weighted tape was lowered into the wells and as it touches the water, the SWL was read out on the ground surface and recorded. The elevations and topographic coordinates were determined with a Geographic Positioning System (GPS). By subtracting the SWL measured of each well from its topographic elevation above mean sea level, the hydraulic head of each well was obtained (Equation 4), which was used to produce the groundwater flow direction map of the area.
(4) |
Where: h is the Hydraulic Head (meter); d is SWL or Depth to water (meter); Z is the Topographic Elevation above mean sea level (meter).
The pumping test involved applying a stress to the aquifers by extracting groundwater from a pumping well and measuring the aquifer response to that stress by monitoring drawdown in function of time. Pumping tests were carried out on 10 boreholes within the study area to determine the aquifer hydraulic properties such as transmissivity and hydraulic conductivity, and incorporated them into appropriate well-flow equations (Equations 5 and 6). A constant rate and recovery test was used in this study through single-well aquifer test: the pumping test and the drawdown measurements were made in the same borehole. The details of the well such as the depth, the radius, the static water level, and the pump setting depth were recorded before starting the pumping. The pumping duration was ranged between 180 minutes to 360 minutes, according to when the steady state was reached. The measuring interval used was 1 minute for the first ten minutes, 5 minutes for the period from 10 minutes to 60 minutes of pumping, 10 minutes from 60 minutes to 120 minutes and finally 30 minutes from 120 minutes until pumping was exhausted. During the aquifer test, the discharge rate of the well and the changes in water levels (drawdown and recovery) in the well were adequately measured.
Transmissivity (T)
In this study, where the abstraction wells were used as observation wells, Cooper-Jacob method was found suitable (Abimiku et al. 2019). The method gives a simpler algebraic solution to the Theis equation and permitted to determine the drawdown without the use of well functions (Okogbue and Omonona, 2013).
(5) |
Where: T is the transmissivity (m2/d); Q is the steady pumping rate (volume per unit time) measured during pumping test; is the drawdown difference per log cycle (meter), obtained by plotting the time against the drawdown in the semilogarithmic plot.
Hydraulic conductivity (K)
It follows that hydraulic conductivity is the ratio of transmissivity to the Aquifer thickness (Equation 6).
(6) |
Where: K is the hydraulic conductivity (m/d); T is transmissivity (m2/d); b is the aquifer thickness (m).
The transmissivity values were obtained from pumping test data while the aquifer thicknesses were deducted from the borehole lithologic logs.
The subsurface conditions in terms of the number of layers in the substrate, the thickness of each layer and the ratio of the resistivity varied according to the layers (Table 1). The results of the quantitative interpretation revealed that typical 4- and 5- geoelectrical subsurface layers characterize the area, generating eight types of resistivity curves. The type curves include 2-KH, 2-HK, 1-HKH, 1-AA, 1-HA, 1-KQH, 1-HAK and 1-KHK (Fig. 3).
S/N | 1st layer | 2nd layer | 3rd layer | 4th layer | 5th layer | Curve type | |||||||||
h1/m | ρ1/Ωm | h2/m | ρ2/Ωm | h3/m | ρ3/Ωm | h4/m | ρ4/Ωm | h5/m | ρ5/Ωm | ||||||
VES 1 | 0.5 | 1,194.4 | 8.4 | 96.5 | 69.9 | 446.9 | ∞ | 341.0 | - | - | HK | ||||
VES 2 | 0.5 | 367.5 | 2.7 | 118.6 | 13.4 | 166.4 | 29.7 | 771.3 | ∞ | 83.7 | HAK | ||||
VES 3 | 0.8 | 404.3 | 4.7 | 48.4 | 15.4 | 1414.1 | ∞ | 5,044.1 | - | - | HA | ||||
VES 4 | 1.8 | 131.0 | 5.2 | 13.1 | 59.2 | 818.9 | ∞ | 265.1 | - | - | HK | ||||
VES 5 | 1.4 | 59.0 | 2.0 | 80.5 | 10.7 | 33.7 | ∞ | 5,378.0 | - | - | KH | ||||
VES 6 | 1.0 | 252.2 | 6.7 | 469.6 | 25.6 | 157.1 | 92.2 | 632.7 | ∞ | 365.1 | KHK | ||||
VES 7 | 1.3 | 77.0 | 3.8 | 531.1 | 2.7 | 159.4 | 12.7 | 72.7 | ∞ | 8846.5 | KQH | ||||
VES 8 | 0.9 | 128.8 | 6.8 | 151.5 | 31.1 | 139.5 | ∞ | 1,734.7 | - | - | KH | ||||
VES 9 | 0.6 | 153.8 | 1.4 | 21.5 | 12.9 | 513.4 | 36.1 | 163.6 | ∞ | 1204.7 | HKH | ||||
VES 10 | 1.0 | 45.0 | 5.1 | 138.6 | 32.5 | 630.2 | ∞ | 5,290.4 | - | - | AA |
The surface layer, averaging 0.98 m thick and ranging from 0.5 m to 1.8 m, is observed in all VES locations. It exhibits a wide variation in resistivity ranging from 45.0 Ωm to 1,194.4 Ωm with an average of 281.23 Ωm and is identified as laterite by correlation with the general lithological log of the area. Meanwhile, the underlying laterite is a layer correlated to reddish brown, sandy clay and clayey sands, with thickness range from 1.4 m to 8.4 m, averaged 4.68 m, and resistivity range of 13.1 Ωm to 531.1 Ωm with an average of 168.94 Ωm (Table 1). The weathered and/or fractured bedrock found in the third and fourth geoelectric layers. In fact, the third layer, showed a range of resistivity between 33.7 Ωm and 1414.1 Ωm with an average value of 499.78 Ωm, with an average thickness of 29.93 m. On the other hand, in the fourth layer, the weathered/fractured zones revealed a resistivity range of (72.7–5378) Ωm and an average thickness of 42.68 m (Table 1).
The measured Static Water Level (SWL), the topographic elevation and the computed hydraulic heads varied among the six selected locations in the study area (Table 2). Indeed, the average hydraulic head values were 212.53 m, 190.1 m, 175 m, 171.8 m and 158.9 m for Dukpa, Passo, Paikon-Kore, Kaida and Koroko locations respectively. However, the Gwagwalada location where only one existing dug well was tested, the hydraulic head value of 211.8 m was correspondingly measured (Table 2). The groundwater flow direction map designed from the hydraulic head values and corresponding coordinates through Surfer 21 software, revealed that the high hydraulic head value is located in the Northwest, through which groundwater in the area flows towards southwest, south and west directions (Fig. 4). Indeed, groundwater flows from regions of high hydraulic head to regions of low hydraulic head.
Location | No. of wells tested | Elevation/m | Static water level/m | Depth of well/m | Hydraulic Head/m |
Dukpa | 9 | 139–224 | 0.8–3.1 | 4–7 | 135.9–282.5 |
Gwagwalada | 1 | 214 | 2.2 | 5.5 | 211.8 |
Passo | 6 | 180–202 | 0.2–3.5 | 4.1–5.7 | 177.5–201.8 |
Paikon Kore | 4 | 174–184 | 0.0–5.5 | 3.2–6 | 169.5–183.9 |
Kaida | 5 | 158–192 | 1.9–2.7 | 4.4–5.7 | 155.7–189.3 |
Koroko | 5 | 154–170 | 1.8–2.8 | 3.0–4.9 | 152.2–167.5 |
The NE-SW groundwater flow direction is shown to be the main one, coinciding with the major principal joint direction, portending that that the groundwater flow direction in the area is structurally controlled. This further explains the overlapping and interwoven nature of geology, structural geology and hydrogeology in relation to groundwater studies.
The groundwater potential was established through comparison of transmissivity and hydraulic conductivity values deducted from pumping test data (Fig. 8). Indeed, the details of aquifer parameters are summarized in the table 3, whereas the transmissivity values ranged from a minimum of 0.35 m2/d to a maximum of 3.63 m2/d at Borehole 5 and Borehole 2 respectively, with an average value of 1.36 m2/d. A contour map of transmissivity in the study area is shown in Fig. 5. Based on the Krasny's transmissivity classification standards by Krasny (1993) (Table 4) the tested aquifers are of two categories namely very low and low. Therefore, they are qualified for small sustainable withdrawal for local water supply.
The transmissivity map shows that the area of very low productivity found in the Northern and Eastern regions of the study area, covering almost 50% while the area of low productivity includes the Central, western and South-western regions. The general borehole lithologic log of the area and the weathered/fractured layer thicknesses obtained from the borehole driller, proved that the subsurface in the study area is made up of lateritic soil, sandy clay or clayey sand, weathered basement, fractured and fresh bedrock (Fig. 6). As such, the aquifers in the area are located within the weathered and/or fractured layer of the bedrock. The weathered/fractured layer thicknesses are ranged from 7.5 meters (borehole 5) to 56.7 meters (borehole 10) with an average value of 18.81 meters (Table 3).
Parameters | Minimum | Maximum | Mean |
Borehole depth (m) | 124 | 200 | 155 |
SWL (m) | 1.2 | 19.4 | 6.8 |
Yield (m3/d) | 82.1 | 319.7 | 158.5 |
Drawdown (m) | 6.6 | 84.6 | 38.7 |
Drawdown per log cycle (m) | 4.8 | 56 | 28.6 |
Weathered/fractured layer thickness (m) | 7.5 | 56.7 | 18.8 |
Transmissivity (m2/d) | 0.35 | 3.6 | 1.36 |
Hydraulic conductivity (m/d) | 0.045 | 0.18 | 0.07 |
Coefficient of T/ m2/d | Class | Magnitude | Groundwater supply potential |
> 1,000 | I | Very high | Withdrawals of great regional importance |
1,000−100 | II | High | Withdrawals of lesser regional importance |
100−10 | III | Intermediate | withdrawal for local water supply |
10−1 | IV | Low | Smaller withdrawal for local water supply |
1−0.1 | V | Very low | withdrawal for local water supply with limited consumption |
< 0.1 | VI | Imperceptible | Source for local water supply is difficult |
Hydraulic conductivity values are ranged from 0.045 m/d to 0.18 m/d, implying that the targeted area is semi-pervious (Table 5). The hydraulic conductivity contour map (Fig. 7) indicates an emphasis of K values in the south-western part of the study area, indicating that the permeability is somewhat more pronounced in this part of the study area. In fact, the high variability in the hydraulic properties, such as transmissivity and hydraulic conductivity from place to another in the targeted area can be subjected to the degree of weathering/fracturing of the geological materials. While assessing the homogeneity of an area, the pair-wise transmissivity revealed that only two cases (BH1 versus BH8 and BH4 versus BH9) found to be less than 5% over 45 (Table 6). Thus, the explored area does not have transmissivity homogeneity.
Km/s | 102 | 101 | 100 | 10−1 | 10−2 | 10−3 | 10−4 | 10−5 | 10−6 | 10−7 | 10−8 | 10−9 | 10−10 |
Relative permeability | Pervious | Semi-pervious | Impervious | ||||||||||
Aquifer | Good | Poor | None | ||||||||||
Consolidated rock | Highly fracturated | Oil reservoir rock | Fresh sandstone | Fresh limestone, dolomite | Fresh granite |
Transmissivity | BH2 | BH3 | BH4 | BH5 | BH6 | BH7 | BH8 | BH9 | BH10 |
BH1 | 74.9 | 45 | 8.1 | 61.4 | 33.4 | 23.2 | 1.3 | 7 | 66.5 |
BH2 | 54.3 | 77 | 90.3 | 83.3 | 67.3 | 75.2 | 76.6 | 24.9 | |
BH3 | 49.5 | 78.8 | 63.3 | 28.3 | 45.7 | 48.8 | 39.2 | ||
BH4 | 58 | 27.5 | 29.5 | 6.9 | 1.3 | 69.3 | |||
BH5 | 42.1 | 70.4 | 60.9 | 58.5 | 87.1 | ||||
BH6 | 48.9 | 32.5 | 28.4 | 77.7 | |||||
BH7 | 24.2 | 28.6 | 56.4 | ||||||
BH8 | 5.7 | 67 | |||||||
BH9 | 68.9 |
The spatial distribution of groundwater potential (Fig. 8) is established on the basis of comparing transmissivity and hydraulic conductivity values. The groundwater potential map shows that the areas where elevated transmissivity values intercept elevated hydraulic conductivity values are located particularly in the south-western portion of the area, thus marking the moderate groundwater potential area of approximately 30 percent. The remaining 70% with low groundwater potential are located in the north and the south-Eastern part of the targeted area (Fig. 8).
The longitudinal conductance values vary from 0.11 Ω−1 to 0.37 Ω−1, with an average value of 0.243 Ω−1(Table 7). Therefore, based on criteria highlighted in the Table 8, the protective capacity for aquifer in the study area can be categorized into two weak and moderate. When comparing various longitudinal conductance, VES 2, VES 3 and VES 10 showed the lowest longitudinal conductance values containing in the interval between 0.1 and 0.19 Ω−1, indicating that they have weak aquifer protective capacity (Table 7). Meanwhile, the longitudinal conductance values computed from VES 1, VES 4, VES 5, VES 6, VES 7, VES 8 and VES 9 are included in the bracket ranging from 0.2 Ω−1 to 0.69 Ω−1, portending moderate aquifer protective capacity (Table 7).
S/N | Longitudinal conductance/Ω−1 |
Protective capacity |
VES 1 | 0.242981 | Moderate |
VES 2 | 0.143161 | Weak |
VES 3 | 0.109976 | Weak |
VES 4 | 0.326328 | Moderate |
VES 6 | 0.326328 | Moderate |
VES 7 | 0.215667 | Moderate |
VES 8 | 0.274811 | Moderate |
VES 9 | 0.314822 | Moderate |
VES 10 | 0.11059 | Weak |
Longitudinal conductance/Ω−1 | Protective capacity |
> 10 | Excellet |
5−10 | Very good |
0.7−4.9 | Good |
0.2−0.69 | Moderate |
0.1−0.19 | Weak |
< 0.1 | Poor |
The longitudinal conductance values were computed through Surfer 21 software to model the aquifer protective capacity pattern in the study area (Fig. 9). The Aquifer protective capacity map shows that approximately 50% of the study area are characterized as area of low protective capacity and tended to be oriented in a NE-SW direction, including the north-eastern, central and south-western as illustrated by purple, blue, green and yellow colors respectively. The remaining 50% are characterized as moderate protective capacity and are located in east, south-east and north-west indicated by dark yellow and orange colors.
Infiltration occurs easily in terrains of weak protective capacity than those with moderate protective capacity. Consequently, the transport of contaminants is barely restricted in such areas. Therefore, the areas of weak aquifer protective capacity are vulnerable to pollution of groundwater resources, mainly of anthropogenic sources which are predominant in the study area.
The resistivity and hydrogeological approaches applied in current study, have unraveled the aquifer hydraulic properties and details on groundwater in the targeted area. The present findings revealed that the Gwagwalada area is characterized by four to five layers, depicting laterite, reddish brown, sandy clay and clayey sands, underlain by weathered/fractured layer and fresh bedrock. The groundwater flows from north-east towards southwest, south and west directions with emphasis in southwest direction, coinciding with the principal joint direction. There is enough evidence to conclude that the aquifer transmissivity in the explored area is classified into low and moderate, whereas protective capacity is subdivided into weak and moderate zones according to standard classifications. Since the aquifer with weak protective capacity are prone to groundwater pollution owing to high infiltration rate, the proper waste management by community is highly recommended. Eventually, this study provided a baseline information for efficient estimation of the amount of groundwater available for exploitation in the study area and to ensure its sustainability.
Acknowledgements: Authors wish to acknowledge the African Water Resources Mobility Network (AWaRMN) for supporting this research through the Intra-African Academic Mobility Programme No. 2019-1973/004-001, which was funded by the European Union.
Abimiku ES, A Dadan-Garba, Adepetu A. 2019. Assessment of groundwater potentials in Ningi Area of Bauchi State, Nigeria. International Journal of Research and Scientific Innovation, 6(6): 155−157.
|
Achichi C, Sennuga SO, Osho-Lagunju B, et al. 2023. Effect of farmers' socioeconomic characteristics on access to agricultural information in Gwagwalada Area Council, Abuja. Discoveries in Agriculture and Food Sciences, 10: 28−47. DOI: 10.14738/dafs.105.14273.
|
Adewumi AJP, Anifowose YB. 2017. Hydrogeologic characterization of Owo and its environs using remote sensing and GIS. Applied Water Science, 7(6): 2987–3000. DOI: 10.1007/s13201-017-0611-8.
|
Amadi AN, Ozoji TM, Aweda AK, et al. 2020. Updates on aquifer attributes of the Benin formation within Eastern Niger Delta, Nigeria using pumping test and hydraulic parameters. Minna Jornal of Geosciences (MJG), 4(1): 37−51.
|
Asfahani J. 2021. Classification of the transmissivity spatial variations of quaternary aquifer in the semi-arid Region Khanasser Valley, Northern Syria. Journal of African Earth Sciences, 182: 104269. DOI: 10.1016/j.jafrearsci.2021.104269.
|
Asije E, Igwe O. 2014. Electrical resistivity investigation for ground water in Parts of Pegi, Federal Capital Territory, Nigeria. IOSR Journal of Applied Geology and Geophysics, 2(6): 27–32. https://www.academia.edu/download/36521752/E02612732.pdf.
|
Chilton JP, Forester SSD. 1993. Hydrogeological characterization and water supply potential of basement aquifers in tropical Africa. Hydrogeology Journal, 3(1): 36−49. DOI: 10.1007/s100400050061.
|
Dan-Hassan MA, Yaya OO. 2007. Application of the hummel (modified schlumberger) method of vertical electrical soundings in groundwater exploration in parts of basement complex of Nigeria. Water Resources, 17: 77−82
|
Dan-hassan MA. 2013. Some Aspect of Hydrogeology of FCT, Abuja, Nigeria. Ph. D. Thesis. Federal University of Technology, Minna: Nigeria.
|
Dan-hassan MA, Olasehinde PI, Amadi AN, et al. 2012. Spatial and temporary distribution of nitrate pollution in groundwater of Abuja, Nigeria. International Journal of chemistry, 4(3): 104−112.
|
Dinneya OC, Adigun A. 2022. Preliminary gold mineralization potential assessment of a site covering parts of Gwagwalada and Abaji Area Council, Federal Capital Territory (Abuja). Communication in Physical Sciences, 8(4).
|
Egbai JC, Emekeme IRE. 2015. Aquifer transmissivity Dar Zarrouk parameters and groundwater flow direction in Abudu, Edo State, Nigeria. International Journal Environmental Science and Technology, 4(3): 628−640.
|
Elimian KO, Mezue S, Musah A, et al. 2020. What are the drivers of recurrent cholera transmission in Nigeria? Evidence from a scoping review. BMC Public Health, 20(1): 1–13. DOI: 10.1186/s12889-020-08521-y
|
Etu-Efeotor JO. 1998. Hydrochemical analysis of surface andgroundwaters of Gwagwalada area of Central Nigeria. Global Journal of Pure and Applied Sciences, 4(2): 153−162.
|
Fetter CW. 2001. Properties of aquifers. Applied Hydrogeology, 4th Edition. Long Grove, Illinois: Waveland Press, Inc.
|
Gleick PH. 1996. Basic water requirements for human activities: Meeting basic needs. Water International, 21(2): 83−92. DOI: 10.1080/02508069608686494.
|
Haque S, Kannaujiya S, Taloor AK, et al. 2020. Identification of groundwater resource zone in the active tectonic region of Himalaya through earth observatory techniques. Groundwater for Sustainable Development, 10: 100337. DOI: 10.1016/j.gsd.2020.100337.
|
Hasan M, Shang Y, Jin W, et al. 2021. Estimation of hydraulic parameters in a hard rock aquifer using integrated surface geoelectrical method and pumping test data in southeast Guangdong, China. Geosciences Journal, 25: 223−242. DOI: 10.1007/s12303-020-0018-7.
|
Hazell JRT, Cratchley CR, Jones CRC. 1992. The hydrogeology of crystalline aquifers in northern Nigeria and geophysical techniques used in their exploration. Geological Society, London, Special Publications, 66(1): 155−182. DOI: 10.1144/GSL.SP.1992.066.01.08.
|
Henriet JP. 1976. Direct application of the Dar-Zarrouk parameters in groundwater survey. Geophysical Prospecting 24(2): 344−353. DOI: 10.1111/j.1365-2478.1976.tb00931.x.
|
Ighalo JO, Adeniyi AG. 2020. A comprehensive review of water quality monitoring and assessment in Nigeria. Chemosphere, 260, 127569. DOI: 10.1016/j.chemosphere.2020.127569.
|
Kasidi S. 2017. Groundwater exploration using electrical resistivity method a case study in Federal Capital Territory (FCT) Abuja. Nigeria. International Journal of Engineering and Applied Sciences (IJEAS), 4(10): 1−8.
|
Lapworth DJ, Boving TB, Kreamer DK, et al. 2022. Groundwater quality: Global threats, opportunities and realising the potential of groundwater. Science of the Total Environment, 811: 152471. DOI: 10.1016/j.scitotenv.2021.152471.
|
Khilchevskyi V, Karamushka V. 2021. Global water resources: Distribution and demand. In Clean Water and Sanitation. Cham: Springer International Publishing: 1−11. DOI: 10.1007/978-3-319-70061-8_101-1.
|
Mahmud S, Hamza S, Irfan M, et al. 2022. Investigation of groundwater resources using electrical resistivity sounding and Dar Zarrouk parameters for Uthal Balochistan, Pakistan. Groundwater for Sustainable Development, 17: 100738. DOI: 10.1016/j.gsd.2022.100738.
|
Mishra RK. 2023. Fresh water availability and its global challenge. British Journal of Multidisciplinary and Advanced Studies, 4(3): 1−78. DOI: 10.37745/bjmas.2022.0208.
|
Naidu S, Gupta G, Shailaja G, et al. 2021. Spatial behavior of the Dar-Zarrouk parameters for exploration and differentiation of water bodies aquifers in parts of Konkan coast of Maharashtra, India. Journal of Coastal Conservation, 25: 1−9. DOI: 10.1007/s11852-021-00807-6.
|
Nazari B, Keshavarz M. 2023. Water population density: Global and regional analysis. Theoretical and Applied Climatology, 153(1): 431−445. DOI: 10.1007/s00704-023-04473-6.
|
Nazario MG, Kuria ZN, Gichaba CM. 2023. Groundwater potential assessment using remote sensing, geographical information system and electrical resistivity methods in crystalline terrain in Kapuri Area, Jubek State, South Sudan. IOSR Journal of Applied Geology and Geophysics (IOSR-JAGG), 11(2): 49−62. DOI: 10.9790/0990-1102014962.
|
Nugraha GU, Nur AA, Pranantya PA, et al. 2022. Analysis of groundwater potential zones using Dar-Zarrouk parameters in Pangkalpinang city, Indonesia. Environment, Development and Sustainability, 25(2): 1876−1898. DOI: 10.1007/s10668-021-02103-7.
|
Offodile ME. 1992. An approach to groundwater study and development in Nigeria. Mecon Geology and Engineering Services Ltd., Jos: 247.
|
Okogbue CO, Omonona OV. 2013. Potentiel en eaux souterraines de la région de socle d'Egbe-Mopa, dans le centre du Nigeria. Hydrological Sciences Journal, 58(4): 826−840. DOI: 10.1080/02626667.2013.775445.
|
Olasehinde PI. 1999. An integrated geologic and geophysical exploration technique for groundwater in the basement complex of west central part of Nigeria. Water Resources, 10: 46−49.
|
Olasehinde PI, Amadi AN, EO Enwedol, et al. 2016. Assessing the groundwater potential in Wuye distrtrct, Federal Capital Territory, Abuja, Nigeria. Development Journal of Science and Technology Research (DJOSTER), 5(1): 67–78.
|
Olayinka AI. 1990. Electromagnetic profiling and resistivity soundings in groundwater investigation near Egbeda-Kabba, Kwara State. Journal of Mining and Geology, 26(2): 243–250.
|
Olorunfemi MO, Fasuyi SA. 1993. Aquifer types and the geoelectric/hydrogeologic characteristic of part of the central basement terrain of Nigeria (Niger State). Journal of African Earth Sciences, 16(3): 309−317. DOI: 10.1016/0899-5362(93)90051-Q.
|
Pal SC, Ghosh C, Chowdhuri I. 2020. Assessment of groundwater potentiality using geospatial techniques in Purba Bardhaman district, West Bengal. Applied Water Science, 10(10): 221. DOI: 10.1007/s13201-020-01302-3.
|
Omada J, Ogoko E, Kelle H, et al. 2024. Water quality and health risk assessment of metals within the vicinity of Gosa dumpsite, Abuja Nigeria. Revista Colombiana de Ciencias Químico-Farmacéuticas, 53(1). DOI: 10.15446/rcciquifa.v53n1.110647.
|
Rizwan MM. 2018. Hydrogeological analysis of shallow groundwater system around bangar area Dewas District Madhya Pradesh, using remote sensing data. Doctoral dissertation, Vikram University: 201. DOI: 10.13140/RG.2.2.15878.32327.
|
Rojanasakul M, Flavelle C, Migliozzi B, et al. 2023. America is using up its groundwater like there's no tomorrow. The New York Times (Digital Edition), NA-NA.
|
Sen Z. 2015. Practical and applied hydrogeology (Elsevier). DOI: 10.1016/B978-0-12-800075-5.05001-9.
|
Sunkari ED, Kore BM, Abioui M. 2021. Hydrogeophysical appraisal of groundwater potential in the fractured basement aquifer of the federal capital territory, Abuja, Nigeria. Results in Geophysical Sciences, 5: 100012. DOI: 10.1016/j.ringps.2021.100012.
|
Umar DUA, Ramli MF, Aris AZ, et al. 2019. Surface water resources management along Hadejia River Basin, northwestern Nigeria. h2Open Journal, 2(1): 184−199. DOI: 10.2166/h2oj.2019.010.
|
Van der Meulen ES, Sutton NB, Van de Ven, et al. 2020. Trends in demand of urban surface water extractions and in situ use functions. Water Resources Management, 34: 4943−4958. DOI: 10.1007/s11269-020-02700-7.
|
Wiederhold H, Kallesøe AJ, Kirsch R, et al. 2021. Geophysical methods help to assess potential groundwater extraction sites. Grundwasser, 26(4): 367−378. DOI: 10.1007/s00767-021-00495-x.
|
Yusuf MA, Abiye TA. 2019. Risks of groundwater pollution in the coastal areas of Lagos, southwestern Nigeria. Groundwater for Sustainable Development, 9: 100222. DOI: 10.1016/j.gsd.2019.100222.
|
Yusuf SN, Ishaku JM, Wakili WM. 2021. Estimation of Dar-Zarrouk parameters and delineation of groundwater potential zones in Karlahi, part of Adamawa Massif, Northeastern Nigeria. Warta Geologi, 47(2): 103−112. DOI: 10.7186/wg472202101.
|
2305-7068/© 2025 Journal of Groundwater Science and Engineering Editorial Office. This is an open access article under the CC BY-NC-ND license (http://creativecommons.org/licenses/by-nc-nd/4.0)
[1] | Stephen Pitchaimani V, Narayanan MSS, Abishek RS, Aswin SK, Jerin Joe RJ, 2024: Delineation of groundwater potential zones using remote sensing and Geographic Information Systems (GIS) in Kadaladi region, Southern India, Journal of Groundwater Science and Engineering, 12, 147-160. doi: 10.26599/JGSE.2024.9280012 |
[2] | Temesgen Mekuriaw Manderso, Yitbarek Andualem Mekonnen, Tadege Aragaw Worku, 2023: Application of GIS based analytical hierarchy process and multicriteria decision analysis methods to identify groundwater potential zones in Jedeb Watershed, Ethiopia, Journal of Groundwater Science and Engineering, 11, 221-236. doi: 10.26599/JGSE.2023.9280019 |
[3] | Edmealem Temesgen, Demelash Wendmagegnehu Goshime, Destaw Akili, 2023: Determination of groundwater potential distribution in Kulfo-Hare watershed through integration of GIS, remote sensing, and AHP in Southern Ethiopia, Journal of Groundwater Science and Engineering, 11, 249-262. doi: 10.26599/JGSE.2023.9280021 |
[4] | Bakuru Anandagajapathi Raju, Palavai Venkateswara Rao, Mangalampalli Subrahmanyam, 2023: Estimating aquifer transmissivity using Dar-Zarrouk parameters to delineate groundwater potential zones in Alluri Seetharama Raju District, Andhra Pradesh, India, Journal of Groundwater Science and Engineering, 11, 116-132. doi: 10.26599/JGSE.2023.9280011 |
[5] | Wondmagegn Taye Abebe, 2022: Evaluation of groundwater resource potential by using water balance model: A case of Upper Gilgel Gibe Watershed, Ethiopia, Journal of Groundwater Science and Engineering, 10, 209-222. doi: 10.19637/j.cnki.2305-7068.2022.03.001 |
[6] | Muthamilselvan A Dr, Sekar Anamika, Ignatius Emmanuel, 2022: Identification of groundwater potential in hard rock aquifer systems using Remote Sensing, GIS and Magnetic Survey in Veppanthattai, Perambalur, Tamilnadu, Journal of Groundwater Science and Engineering, 10, 367-380. doi: 10.19637/j.cnki.2305-7068.2022.04.005 |
[7] | Wondesen Fikade Niway, Dagnachew Daniel Molla, Tarun Kumar Lohani, 2022: Holistic approach of GIS based Multi-Criteria Decision Analysis (MCDA) and WetSpass models to evaluate groundwater potential in Gelana watershed of Ethiopia, Journal of Groundwater Science and Engineering, 10, 138-152. doi: 10.19637/j.cnki.2305-7068.2022.02.004 |
[8] | Rustadi, I Gede Boy Darmawan, Nandi Haerudin, Agus Setiawan, Suharno, 2022: Groundwater exploration using integrated geophysics method in hard rock terrains in Mount Betung Western Bandar Lampung, Indonesia, Journal of Groundwater Science and Engineering, 10, 10-18. doi: 10.19637/j.cnki.2305-7068.2022.01.002 |
[9] | Van Hoang Nguyen, 2021: Determination of groundwater solute transport parameters in finite element modelling using tracer injection and withdrawal testing data, Journal of Groundwater Science and Engineering, 9, 292-303. doi: 10.19637/j.cnki.2305-7068.2021.04.003 |
[10] | Muhammad Juandi, Islami Nur, 2021: Prediction criteria for groundwater potential zones in Kemuning District, Indonesia using the integration of geoelectrical and physical parameters, Journal of Groundwater Science and Engineering, 9, 12-19. doi: 10.19637/j.cnki.2305-7068.2021.01.002 |
[11] | Marios C Kirlas, 2021: Assessment of porous aquifer hydrogeological parameters using automated groundwater level measurements in Greece, Journal of Groundwater Science and Engineering, 9, 269-278. doi: 10.19637/j.cnki.2305-7068.2021.04.001 |
[12] | Zhi-yuan LIU, Ding TAN, Zhi-bin CHEN, Yun-fei WEI, Quan CHAI, Xiao-hang CHEN, 2020: Study on multiple induced polarization parameters in groundwater exploration in Bashang poverty alleviation area of Heibei Province, China, Journal of Groundwater Science and Engineering, 8, 274-280. doi: 10.19637/j.cnki.2305-7068.2020.03.007 |
[13] | Dinagarapandi Pandi, Saravanan Kothandaraman, Mohan Kuppusamy, 2020: Delineation of potential groundwater zones based on multicriteria decision making technique, Journal of Groundwater Science and Engineering, 8, 180-194. doi: 10.19637/j.cnki.2305-7068.2020.02.009 |
[14] | Mehmood Qaisar, Arshad Muhammad, Rizwan Muhammad, Hamid Shanawar, Mehmood Waqas, Ansir Muneer Muhammad, Irfan Muhammad, Anjum Lubna, 2020: Integration of geoelectric and hydrochemical approaches for delineation of groundwater potential zones in alluvial aquifer, Journal of Groundwater Science and Engineering, 8, 366-380. doi: 10.19637/j.cnki.2305-7068.2020.04.007 |
[15] | Nouayti Abderrahime, Khattach Driss, Hilali Mohamed, Nouayti Nordine, 2019: Mapping potential areas for groundwater storage in the High Guir Basin (Morocco):Contribution of remote sensing and geographic information system, Journal of Groundwater Science and Engineering, 7, 309-322. doi: DOI: 10.19637/j.cnki.2305-7068.2019.04.002 |
[16] | SADIKI Moulay Lhassan, EL MANSOURI Bouabid, BENSEDDIK Badr, CHAO Jamal, KILI Malika, EL MEZOUARY Lhoussaine, 2019: Improvement of groundwater resources potential by artificial recharge technique: A case study of Charf El Akab aquifer in the Tangier region, Morocco, Journal of Groundwater Science and Engineering, 7, 224-236. doi: DOI: 10.19637/j.cnki.2305-7068.2019.03.003 |
[17] | LIU Yu, CHENG Yan-pei, GE Li-qiang, 2018: Analysis on exploitation status, potential and strategy of groundwater resources in the five countries of Central Asia, Journal of Groundwater Science and Engineering, 6, 49-57. doi: 10.19637/j.cnki.2305-7068.2018.01.006 |
[18] | WU Ting-wen, WANG Li-huan, YANG Xiang-kui, 2017: Evaluation of groundwater potential and eco-geological environment quality in Sanjiang Plain of Heilongjiang Province, Journal of Groundwater Science and Engineering, 5, 193-201. |
[19] | LIU Min, NIE Zhen-long, WANG Jin-zhe, WANG Li-fang, TIAN Yan-liang, 2016: An assessment of the carrying capacity of groundwater resources in North China Plain region–Analysis of potential for development, Journal of Groundwater Science and Engineering, 4, 174-187. |
[20] | LIU Li-jun, LIU Zhi-gang, LI Dou, ZHANG Shao-cai, CUI Qiu-ping, WANG Juan, 2014: Evaluation of groundwater supply capacity for agricultural drought emergency relief of Hebei Plain, Journal of Groundwater Science and Engineering, 2, 36-45. |
JGSE-ScholarOne Manuscript Launched on June 1, 2024.
S/N | 1st layer | 2nd layer | 3rd layer | 4th layer | 5th layer | Curve type | |||||||||
h1/m | ρ1/Ωm | h2/m | ρ2/Ωm | h3/m | ρ3/Ωm | h4/m | ρ4/Ωm | h5/m | ρ5/Ωm | ||||||
VES 1 | 0.5 | 1,194.4 | 8.4 | 96.5 | 69.9 | 446.9 | ∞ | 341.0 | - | - | HK | ||||
VES 2 | 0.5 | 367.5 | 2.7 | 118.6 | 13.4 | 166.4 | 29.7 | 771.3 | ∞ | 83.7 | HAK | ||||
VES 3 | 0.8 | 404.3 | 4.7 | 48.4 | 15.4 | 1414.1 | ∞ | 5,044.1 | - | - | HA | ||||
VES 4 | 1.8 | 131.0 | 5.2 | 13.1 | 59.2 | 818.9 | ∞ | 265.1 | - | - | HK | ||||
VES 5 | 1.4 | 59.0 | 2.0 | 80.5 | 10.7 | 33.7 | ∞ | 5,378.0 | - | - | KH | ||||
VES 6 | 1.0 | 252.2 | 6.7 | 469.6 | 25.6 | 157.1 | 92.2 | 632.7 | ∞ | 365.1 | KHK | ||||
VES 7 | 1.3 | 77.0 | 3.8 | 531.1 | 2.7 | 159.4 | 12.7 | 72.7 | ∞ | 8846.5 | KQH | ||||
VES 8 | 0.9 | 128.8 | 6.8 | 151.5 | 31.1 | 139.5 | ∞ | 1,734.7 | - | - | KH | ||||
VES 9 | 0.6 | 153.8 | 1.4 | 21.5 | 12.9 | 513.4 | 36.1 | 163.6 | ∞ | 1204.7 | HKH | ||||
VES 10 | 1.0 | 45.0 | 5.1 | 138.6 | 32.5 | 630.2 | ∞ | 5,290.4 | - | - | AA |
Location | No. of wells tested | Elevation/m | Static water level/m | Depth of well/m | Hydraulic Head/m |
Dukpa | 9 | 139–224 | 0.8–3.1 | 4–7 | 135.9–282.5 |
Gwagwalada | 1 | 214 | 2.2 | 5.5 | 211.8 |
Passo | 6 | 180–202 | 0.2–3.5 | 4.1–5.7 | 177.5–201.8 |
Paikon Kore | 4 | 174–184 | 0.0–5.5 | 3.2–6 | 169.5–183.9 |
Kaida | 5 | 158–192 | 1.9–2.7 | 4.4–5.7 | 155.7–189.3 |
Koroko | 5 | 154–170 | 1.8–2.8 | 3.0–4.9 | 152.2–167.5 |
Parameters | Minimum | Maximum | Mean |
Borehole depth (m) | 124 | 200 | 155 |
SWL (m) | 1.2 | 19.4 | 6.8 |
Yield (m3/d) | 82.1 | 319.7 | 158.5 |
Drawdown (m) | 6.6 | 84.6 | 38.7 |
Drawdown per log cycle (m) | 4.8 | 56 | 28.6 |
Weathered/fractured layer thickness (m) | 7.5 | 56.7 | 18.8 |
Transmissivity (m2/d) | 0.35 | 3.6 | 1.36 |
Hydraulic conductivity (m/d) | 0.045 | 0.18 | 0.07 |
Coefficient of T/ m2/d | Class | Magnitude | Groundwater supply potential |
> 1,000 | I | Very high | Withdrawals of great regional importance |
1,000−100 | II | High | Withdrawals of lesser regional importance |
100−10 | III | Intermediate | withdrawal for local water supply |
10−1 | IV | Low | Smaller withdrawal for local water supply |
1−0.1 | V | Very low | withdrawal for local water supply with limited consumption |
< 0.1 | VI | Imperceptible | Source for local water supply is difficult |
Km/s | 102 | 101 | 100 | 10−1 | 10−2 | 10−3 | 10−4 | 10−5 | 10−6 | 10−7 | 10−8 | 10−9 | 10−10 |
Relative permeability | Pervious | Semi-pervious | Impervious | ||||||||||
Aquifer | Good | Poor | None | ||||||||||
Consolidated rock | Highly fracturated | Oil reservoir rock | Fresh sandstone | Fresh limestone, dolomite | Fresh granite |
Transmissivity | BH2 | BH3 | BH4 | BH5 | BH6 | BH7 | BH8 | BH9 | BH10 |
BH1 | 74.9 | 45 | 8.1 | 61.4 | 33.4 | 23.2 | 1.3 | 7 | 66.5 |
BH2 | 54.3 | 77 | 90.3 | 83.3 | 67.3 | 75.2 | 76.6 | 24.9 | |
BH3 | 49.5 | 78.8 | 63.3 | 28.3 | 45.7 | 48.8 | 39.2 | ||
BH4 | 58 | 27.5 | 29.5 | 6.9 | 1.3 | 69.3 | |||
BH5 | 42.1 | 70.4 | 60.9 | 58.5 | 87.1 | ||||
BH6 | 48.9 | 32.5 | 28.4 | 77.7 | |||||
BH7 | 24.2 | 28.6 | 56.4 | ||||||
BH8 | 5.7 | 67 | |||||||
BH9 | 68.9 |
S/N | Longitudinal conductance/Ω−1 |
Protective capacity |
VES 1 | 0.242981 | Moderate |
VES 2 | 0.143161 | Weak |
VES 3 | 0.109976 | Weak |
VES 4 | 0.326328 | Moderate |
VES 6 | 0.326328 | Moderate |
VES 7 | 0.215667 | Moderate |
VES 8 | 0.274811 | Moderate |
VES 9 | 0.314822 | Moderate |
VES 10 | 0.11059 | Weak |
Longitudinal conductance/Ω−1 | Protective capacity |
> 10 | Excellet |
5−10 | Very good |
0.7−4.9 | Good |
0.2−0.69 | Moderate |
0.1−0.19 | Weak |
< 0.1 | Poor |
S/N | 1st layer | 2nd layer | 3rd layer | 4th layer | 5th layer | Curve type | |||||||||
h1/m | ρ1/Ωm | h2/m | ρ2/Ωm | h3/m | ρ3/Ωm | h4/m | ρ4/Ωm | h5/m | ρ5/Ωm | ||||||
VES 1 | 0.5 | 1,194.4 | 8.4 | 96.5 | 69.9 | 446.9 | ∞ | 341.0 | - | - | HK | ||||
VES 2 | 0.5 | 367.5 | 2.7 | 118.6 | 13.4 | 166.4 | 29.7 | 771.3 | ∞ | 83.7 | HAK | ||||
VES 3 | 0.8 | 404.3 | 4.7 | 48.4 | 15.4 | 1414.1 | ∞ | 5,044.1 | - | - | HA | ||||
VES 4 | 1.8 | 131.0 | 5.2 | 13.1 | 59.2 | 818.9 | ∞ | 265.1 | - | - | HK | ||||
VES 5 | 1.4 | 59.0 | 2.0 | 80.5 | 10.7 | 33.7 | ∞ | 5,378.0 | - | - | KH | ||||
VES 6 | 1.0 | 252.2 | 6.7 | 469.6 | 25.6 | 157.1 | 92.2 | 632.7 | ∞ | 365.1 | KHK | ||||
VES 7 | 1.3 | 77.0 | 3.8 | 531.1 | 2.7 | 159.4 | 12.7 | 72.7 | ∞ | 8846.5 | KQH | ||||
VES 8 | 0.9 | 128.8 | 6.8 | 151.5 | 31.1 | 139.5 | ∞ | 1,734.7 | - | - | KH | ||||
VES 9 | 0.6 | 153.8 | 1.4 | 21.5 | 12.9 | 513.4 | 36.1 | 163.6 | ∞ | 1204.7 | HKH | ||||
VES 10 | 1.0 | 45.0 | 5.1 | 138.6 | 32.5 | 630.2 | ∞ | 5,290.4 | - | - | AA |
Location | No. of wells tested | Elevation/m | Static water level/m | Depth of well/m | Hydraulic Head/m |
Dukpa | 9 | 139–224 | 0.8–3.1 | 4–7 | 135.9–282.5 |
Gwagwalada | 1 | 214 | 2.2 | 5.5 | 211.8 |
Passo | 6 | 180–202 | 0.2–3.5 | 4.1–5.7 | 177.5–201.8 |
Paikon Kore | 4 | 174–184 | 0.0–5.5 | 3.2–6 | 169.5–183.9 |
Kaida | 5 | 158–192 | 1.9–2.7 | 4.4–5.7 | 155.7–189.3 |
Koroko | 5 | 154–170 | 1.8–2.8 | 3.0–4.9 | 152.2–167.5 |
Parameters | Minimum | Maximum | Mean |
Borehole depth (m) | 124 | 200 | 155 |
SWL (m) | 1.2 | 19.4 | 6.8 |
Yield (m3/d) | 82.1 | 319.7 | 158.5 |
Drawdown (m) | 6.6 | 84.6 | 38.7 |
Drawdown per log cycle (m) | 4.8 | 56 | 28.6 |
Weathered/fractured layer thickness (m) | 7.5 | 56.7 | 18.8 |
Transmissivity (m2/d) | 0.35 | 3.6 | 1.36 |
Hydraulic conductivity (m/d) | 0.045 | 0.18 | 0.07 |
Coefficient of T/ m2/d | Class | Magnitude | Groundwater supply potential |
> 1,000 | I | Very high | Withdrawals of great regional importance |
1,000−100 | II | High | Withdrawals of lesser regional importance |
100−10 | III | Intermediate | withdrawal for local water supply |
10−1 | IV | Low | Smaller withdrawal for local water supply |
1−0.1 | V | Very low | withdrawal for local water supply with limited consumption |
< 0.1 | VI | Imperceptible | Source for local water supply is difficult |
Km/s | 102 | 101 | 100 | 10−1 | 10−2 | 10−3 | 10−4 | 10−5 | 10−6 | 10−7 | 10−8 | 10−9 | 10−10 |
Relative permeability | Pervious | Semi-pervious | Impervious | ||||||||||
Aquifer | Good | Poor | None | ||||||||||
Consolidated rock | Highly fracturated | Oil reservoir rock | Fresh sandstone | Fresh limestone, dolomite | Fresh granite |
Transmissivity | BH2 | BH3 | BH4 | BH5 | BH6 | BH7 | BH8 | BH9 | BH10 |
BH1 | 74.9 | 45 | 8.1 | 61.4 | 33.4 | 23.2 | 1.3 | 7 | 66.5 |
BH2 | 54.3 | 77 | 90.3 | 83.3 | 67.3 | 75.2 | 76.6 | 24.9 | |
BH3 | 49.5 | 78.8 | 63.3 | 28.3 | 45.7 | 48.8 | 39.2 | ||
BH4 | 58 | 27.5 | 29.5 | 6.9 | 1.3 | 69.3 | |||
BH5 | 42.1 | 70.4 | 60.9 | 58.5 | 87.1 | ||||
BH6 | 48.9 | 32.5 | 28.4 | 77.7 | |||||
BH7 | 24.2 | 28.6 | 56.4 | ||||||
BH8 | 5.7 | 67 | |||||||
BH9 | 68.9 |
S/N | Longitudinal conductance/Ω−1 |
Protective capacity |
VES 1 | 0.242981 | Moderate |
VES 2 | 0.143161 | Weak |
VES 3 | 0.109976 | Weak |
VES 4 | 0.326328 | Moderate |
VES 6 | 0.326328 | Moderate |
VES 7 | 0.215667 | Moderate |
VES 8 | 0.274811 | Moderate |
VES 9 | 0.314822 | Moderate |
VES 10 | 0.11059 | Weak |
Longitudinal conductance/Ω−1 | Protective capacity |
> 10 | Excellet |
5−10 | Very good |
0.7−4.9 | Good |
0.2−0.69 | Moderate |
0.1−0.19 | Weak |
< 0.1 | Poor |